Processes
The focus of this activity is to better understand and represent processes and physical feedbacks that are relevant for weather and climate in Central Europe and the Alpine region in particular. These studies investigate processes across spatial scales ranging from O(100 m) to O(1000 km).
Pseudo-global-warming (PGW) simulations are a powerful tool to study the importance of different processes associated with climate change. The method was first introduced by Schär et al. 1996. In a PGW simulation, a regional climate model is forced with a process of interest taken from a global climate simulation (Fig. 1). As an example, we used PGW simulations to show that non-homogeneous changes in the atmospheric stratification have a crucial influence on the structure of climate change in Europe during summer (e.g. Kröner et al. 2017).
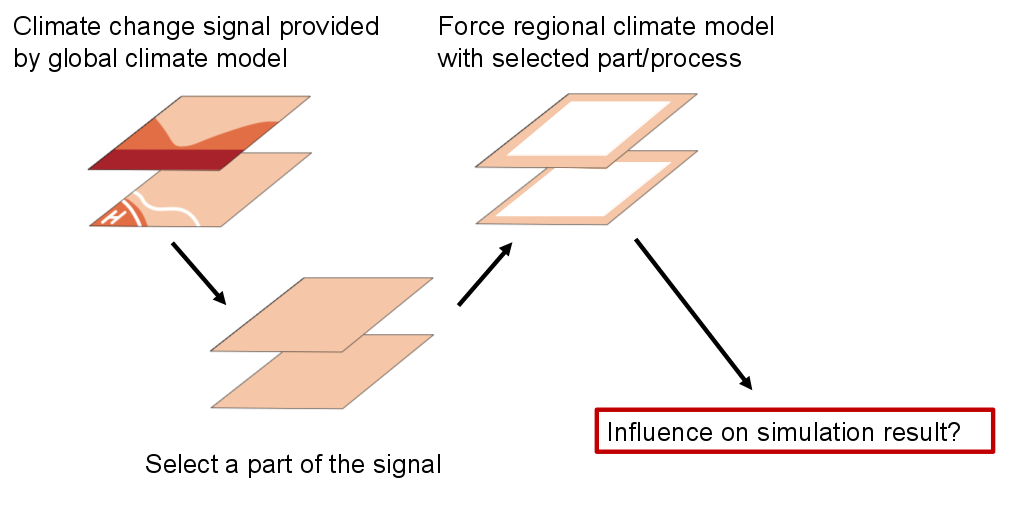
In recent studies, the convergence of domain-averaged and integrated properties related to a large ensemble of convective cells (hence bulk convergence) at kilometer-scale resolutions was demonstrated in both idealized (Panosetti et al., 2018a) and real-case (Langhans et al., 2012; Panosetti et al., 2018b) simulations. These results prove that the mean flow properties and the feedbacks between convective clouds and the large-scale environment are relatively insensitive to further refinement of the mesh grid, and thus support the use of kilometer-scale resolutions in climate models.
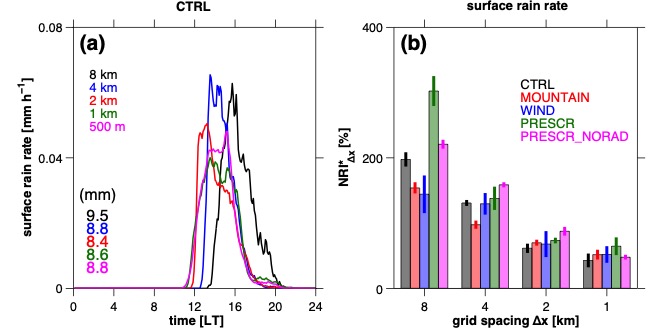
The goal of this activity is to understand the key parameters that determine the characteristics of moist convection in the European summer climate and to investigate the sensitivity of these parameters to changes in future climates. This is done by reducing the complexity of the full earth system in idealized studies. Different settings covering present-day and future climates are addressed. In addition, the role of orography and soil-moisture inhomogeneity in triggering and organizing moist convection is investigated within idealized settings. The knowledge gained from these studies flows back into real-case climate studies.
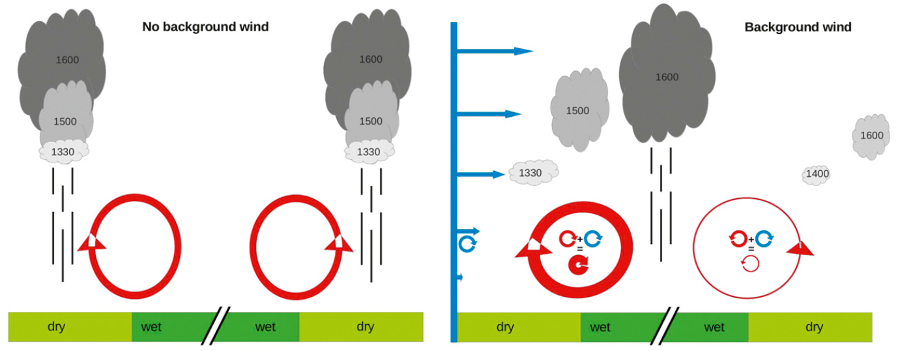
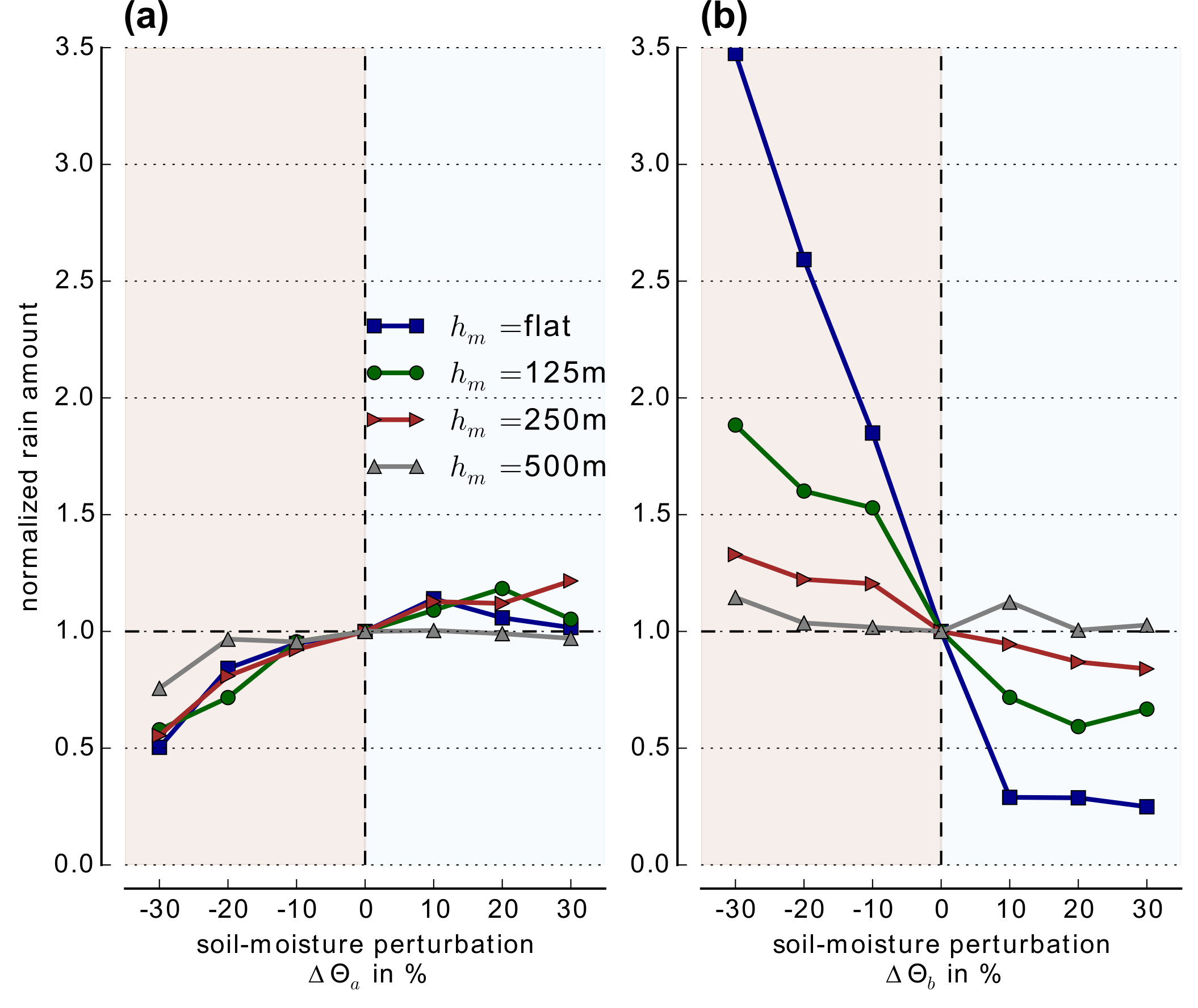
The COSMO model is validated using satellite products from GERB, SEVIRI and AVHRR sensors, a raingauge-based precipitation data set and temperature measurements from surface stations. The validation is done for 12 km (convection-parameterizing model) simulations as well as 2 km (convection-resolving model) simulations. Radiative transfer models are used to generate synthetic satellite radiances. These synthetic satellite images are then compared to the observed satellite measurements. This approach benefits from a direct comparison of model states with observed states, without any assumptions about the observed atmosphere.

Frei et al. 2018, Future snowfall in the Alps: projections based on the EURO-CORDEX regional climate models. The Cryosphere.
Schär and Kröner 2017, Sequential Factor Separation for the Analysis of Numerical Model Simulations. Journal of the Atmospheric Sciences.
Kröner et al. 2017, Separating climate change signals into thermodynamic, lapse-rate and circulation effects: theory and application to the European summer climate. Climate Dynamics.
Schär, C., C. Frei, D. Luthi, and H. C. Davies, 1996: Surrogate climate-change scenarios for regional climate models. Geophysical Research Letters.
Panosetti, D., L. Schlemmer, and C. Schär, 2018a: Convergence behavior of idealized convection-resolving simulations of summertime deep convection over land. Clim. Dyn., external pagehttps://doi.org/10.1007/s00382-018-4229-9call_made
Panosetti, D., L. Schlemmer, and C. Schär, 2018b: Bulk and structural convergence at convection-resolving scales in real-case simulations of summertime moist convection over land. Quart. J. Roy. Meteor. Soc., under review.
Imamovic, A., Schlemmer, L., & Schär, C. (2017). Collective impacts of orography and soil moisture on the soil moisture‐precipitation feedback. Geophysical Research Letters, 44, 11,682–11,691. external pagehttps://doi.org/10.1002/2017GL075657call_made
Panosetti, D., S. Böing, L. Schlemmer, and J. Schmidli (2016): Idealized large-eddy and convection-resolving simulations of moist convection over mountainous terrain. J. Atmos. Sci., 73, 4021–4041, external pagehttps://doi.org/10.1175/JAS-D-15-0341.1call_made
Keller, M., Fuhrer, O., Schmidli, J., Stengel, M., Stöckli, R. and Schär, C. (2015): Evaluation of convection-resolving models using satellite data: The diurnal cycle of summer convection over the Alps, Meteorologische Zeitschrift, external pagedoi: http://doi.org/10.1127/metz/2015/0715call_made
Froidevaux, P., L. Schlemmer, J. Schmidli, W. Langhans, and C. Schär (2014): Influence of the background wind on the local soil-moisture precipitation feedback, J. Atmos. Sc., external pagedoi: http://dx.doi.org/10.1175/JAS-D-13-0180.1call_made
Langhans, W., J. Schmidli, C. Schär (2012) Bulk Convergence of cloud-resolving simulations of moist convection over complex terrain, J. Atmos. Sc., external pagedoi: http://dx.doi.org/10.1175/JAS-D-11-0252.1call_made
Schlemmer, L., C. Hohenegger, J. Schmidli, C. Bretherton, and C. Schär (2011): An idealized cloud-resolving framework for the study of summertime midlatitude diurnal convection over land, J. Atmos. Sci., external pagedoi: http://dx.doi.org/10.1175/2010JAS3640.1call_made